Sergio Loyola, Claudia Cu00e1rcamo, Francisca Concha, Cristian Becerra, Luis Pouchucq*
Volume1-Issue4
Dates: Received: 2020-07-24 | Accepted: 2020-08-06 | Published: 2020-08-07
Pages: 055-063
Abstract
Finding of vegetal species suitable for ecologic restoration in arid climates is a primary goal of most of the governmental and private companies for fighting against the desertification and the recovery of contaminated soils. The Prosopis genus, a desert woody leguminous, with a wide distribution around the world, represents a very interesting source of highly tolerant and adaptable trees for revegetation and bioremediation of soils contaminated with metal and metalloids (metal(loid)s). The aim of the present work was to evaluate the South American representative of this genus, P. alba, as a possible solution for restoration of soils contaminated with mercury and arsenic. For this, we assessed possible tolerance mechanisms against both metal (loid) s by means of the evaluation of bioaccumulation behaviors and expression changes in metal (loid) tolerance genes. The results revealed that P. alba was able to tolerate high metal (loid) concentrations, related with the accumulation of high quantities of arsenic and mercury in the roots, with bioaccumulation factors of 2, 8 and 3, 1 respectively. Moreover, changes in the expression levels of the gene codifying for the metal transporter NRAMP2b was also observed after the exposure to the metal (loid) s, decreasing ten times for arsenic and three times for mercury. All of these results revealed the existence of cellular mechanisms that allow P. Alba to tolerate and accumulate high quantities of arsenic and mercury diluted into the substrate, making it a promising prospect for the treatment of contaminated soils.
FullText HTML
FullText PDF
DOI: 10.37871/jels1121
Certificate of Publication
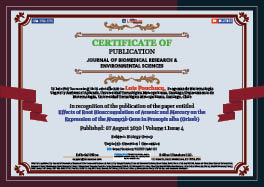
Copyright
© 2020 Loyola S, et al. Distributed under Creative Commons CC-BY 4.0
How to cite this article
Loyola S, Cárcamo C, Concha F, Becerra C, Pouchucq L. Effects of Root Bioaccumulation of Arsenic and Mercury on the Expression of the Nramp2b Gene in Prosopis alba (Griseb). J Biomed Res Environ Sci. 2020 Aug 07; 1(4): 055-063. doi: 10.37871/jels1121, Article ID: jels1121
Subject area(s)
University/Institute
References
- Villagra P, Vilela A, Giordano C, Alvarez J. Ecophysiology of prosopis species from the arid lands of Argentina: What do we know about adaptation to stressful environments. Desert Plants Biology and Biotechnology. Springer-Verlag. Berlin Heidelberg. 2010. DOI: https://doi.org/10.1007/978-3-642-02550-1
- Shackleton R, Le Maitre D, Pasiecznik N, Richardson D. Prosopis: A global assessment of the biogeography, benefits, impacts and management of one of the world’s worst woody invasive plant taxa. AoB Plants. 2014; 6. DOI: https://doi.org/10.1093/aobpla/plu027
- McRostie V, Gayo E, Santoro C, De Pol-Holz R, Latorre C. The pre-columbian introduction and dispersal of Algarrobo (Prosopis, Section Algarobia) in the Atacama Desert of northern Chile. PLoS One. 2017; 12: e0181759. DOI: https://doi.org/10.1371/journal.pone.0181759
- Pasiecznik N, Felker P, Harris P, Harsh L, Cruz G, Tewari J, et al. The Prosopis juliflora - Prosopis pallida Complex: A Monograph, Hdra; Coventry UK. 2001; 172. https://bit.ly/2ENTqdZ
- Geesing D, Felker P, Bingham R. Influence of mesquite (Prosopis glandulosa) on soil nitrogen and carbon development: Implications for global carbon sequestration. J Arid Environ. 2000; 47: 157-180. DOI: https://doi.org/10.1006/jare.2000.0661
- Saini P, Khan S, Baunthiyal M, Sharma V. Organ-wise accumulation of fluoride in Prosopis juliflora and its potential for phytoremediation of fluoride contaminated soil. Chemosphere. 2012; 89: 633-635. DOI: https://doi.org/10.1016/j.chemosphere.2012.05.034
- Arias J, Peralta-Videa J, Ellzey J, Ren M, Viveros M, Gardea-Torresdey J, et al. Effects of Glomus deserticola inoculation on Prosopis: Enhancing chromium and lead uptake and translocation as confirmed by X-ray mapping, ICP-OES and TEM techniques. Environ. Exp. Bot. 2010; 68: 139-148. DOI: https://doi.org/10.1016/j.envexpbot.2009.08.009
- Arias J, Peralta-Videa J, Ellzey J, Viveros M, Ren M, Mokgalaka-Matlala N, et al. Plant growth and metal distribution in tissues of Prosopis Juliflora-velutina grown on chromium contaminated soil in the presence of Glomus deserticola. Environ Sci Technol. 2010; 44: 7272-7279. DOI: https://doi.org/10.1021/es1008664
- Buendía-González L, Orozco-Villafuerte J, Cruz-Sosa F, Barrera-Díaz C, Vernon-Carter E. Prosopis laevigata a potential chromium (VI) and cadmium (II) hyperaccumulator desert plant. Bioresour. Technol. 2010; 101: 5862-5867. DOI: https://doi.org/10.1016/j.biortech.2010.03.027
- Aldrich M, Gardea-Torresdey J, Peralta-Videa J, Parsons J. Uptake and reduction of Cr (VI) to Cr (III) by mesquite (Prosopis spp.): Chromate-plant interaction in hydroponics and solid media studied using XAS. Environ Sci Technol. 2003; 37: 1859-1864. DOI: https://doi.org/10.1021/es0208916
- Varun M, D’Souza R, Pratas J, Paul M. Phytoextraction potential of Prosopis juliflora (Sw.) DC. with specific reference to lead and cadmium. Bull. Environ. Contam. Toxicol. 2011; 87: 45-49. DOI: https://doi.org/10.1007/s00128-011-0305-0
- Aldrich MV, Peralta-Videa JR, Parsons JG, Gardea-Torresdey JL. Examination of arsenic (III) and (V) uptake by the desert plant species mesquite (Prosopis spp.) using X-ray absorption spectroscopy. Sci Total Environ. 2007; 379: 249-255. DOI: https://doi.org/10.1016/j.scitotenv.2006.08.053
- Ferreyra L, Bessega C, Vilardi JC, Saidman B. Consistency of population genetics parameters estimated from isozyme and RAPDs dataset in species of genus Prosopis (Leguminosae, Mimosoideae). Genetica. 2007; 131: 217–230. DOI: https://doi.org/10.1007/s10709-006-9132-3
- Pasiecznik N, Harris PJC, Smith JS. Identifying Tropical Prosopis Species: A Field Guide. Hdra; Coventry UK. 2004; 44: 1-30. https://bit.ly/2DM8kkk
- Morales M, Oakley L, Sartori A, Mogni V, Atahuachi M, Vanni R, et al. Diversity and conservation of legumes in the Gran Chaco and biogeograpical inferences. PLoS ONE. 2019; 14: e0220151. DOI: https://doi.org/10.1371/journal.pone.0220151
- Meloni D, Gulotta M, Silva D, Arraiza M. Effects of salt stress on germination, seedling growth, osmotic adjustment, and chlorophyll fluorescence in Prosopis alba G. Rev. La Fac. Ciencias Agrar. 2019; 51: 69-78. https://bit.ly/3a2q6vO
- Pulford I, Watson C. Phytoremediation of heavy metal-contaminated land by trees - A review Environ Int. 2003; 29: 529-540. DOI: https://doi.org/10.1016/S0160-4120(02)00152-6
- Memon AR, Schröder P. Implications of metal accumulation mechanisms to phytoremediation. Environ Sci Pollut Res Int. 2009; 16: 162-175. DOI: https://doi.org/10.1007/s11356-008-0079-z
- Krämer U, Talke IN, Hanikenne M. Transition metal transport. FEBS Lett. 2007; 581: 2263-2272. DOI: https://doi.org/10.1016/j.febslet.2007.04.010
- Clemens S. Toxic metal accumulation, responses to exposure and mechanisms of tolerance in plants. Biochimie. 2006; 88: 1707-1719. DOI: https://doi.org/10.1016/j.biochi.2006.07.003
- Yang X, Feng Y, He Z, Stoffella P. Molecular mechanisms of heavy metal hyperaccumulation and phytoremediation. J Trace Elem Med Biol. 2005; 18: 339-353. DOI: https://doi.org/10.1016/j.jtemb.2005.02.007.
- Finney DJ. Probit Analysis, 2nd ed., Cambridge University Press, New York, 1952. DOI: https://doi.org/10.1002/jps.3030411125
- Gei V, Erskine PD, Harris HH, Echevarria G, Mesjasz-Przybyłowicz J, Barnabas A, et al. Tools for the discovery of hyperaccumulator plant species and understanding their ecophysiology. Agromining: Farming for Metals. 2018; 117-133. DOI: https://doi.org/10.1007/978-3-319-61899-9
- Fellin M, Negri M, Zanuttini R. Multi-elemental analysis of wood waste using Energy Dispersive X-ray Fluorescence (ED-XRF) analyzer. Eur J Wood & Wood Prod. 2014; 72: 199-211. DOI: https://doi.org/10.1007/s00107-013-0766-4
- Bruinsma J. The quantitative analysis of chlorophylls a and b in plant extracts, photochem. Photobiol. 1963; 2: 241-249. DOI: https://doi.org/10.1111/j.1751-1097.1963.tb08220.x
- Torales SL, Rivarola M, Pomponio MF, Gonzalez S, Acuña CV, Fernández P, et al, De novo assembly and characterization of leaf transcriptome for the development of functional molecular markers of the extremophile multipurpose tree species Prosopis alba. BMC Genomics. 2013; 14: 705. DOI: https://doi.org/10.1186/1471-2164-14-705
- Micol-Ponce R, Sarmiento-Mañús R, Ruiz-Bayón A, Montacié C, Sáez-Vasquez J, Ponce M. Arabidopsis ribosomal RNA processing is required for 18s rRNA maturation. Plant Cell. 2018; 30: 2855-2872. DOI: https://doi.org/10.1105/tpc.18.00245
- Wang X, Fu Y, Ban L, Wang Z, Feng G, Li J, et al. Selection of reliable reference genes for quantitative real-time RT-PCR in alfalfa. Genes Genet Syst. 2015; 90: 175-180. DOI: https://doi.org/10.1266/ggs.90.175
- Schmittgen TD, Zakrajsek BA, Mills AG, Gorn V, Singer MJ, Reed MW. Quantitative reverse transcription-polymerase chain reaction to study mRNA decay: Comparison of endpoint and real-time methods. Anal Biochem. 2000; 285: 194-204. DOI: https://doi.org/10.1006/abio.2000.4753
- Lin Y, Aarts MG. The molecular mechanism of zinc and cadmium stress response in plants. Cell Mol Life Sci. 2012; 69: 3187-3206. DOI: https://doi.org/10.1007/s00018-012-1089-z
- Qin L, Han P, Chen L, Walk T, Li Y, Hu X, et al. Genome-wide identification and expression analysis of NRAMP Family Genes in Soybean (Glycine Max L.). Front Plant Sci. 2017; 8: 1436. DOI: https://doi.org/10.3389/fpls.2017.01436
- Thomine S, Wang R, Ward JM, Crawford N, Schroeder JI. Cadmium and iron transport by members of a plant metal transporter family in Arabidopsis with homology to Nramp genes. PNAS. 2000; 97: 4991-4996. DOI: https://doi.org/10.1073/pnas.97.9.4991
- Bereczky Z, Wang H, Schubert V, Ganal M, Bauer P. Differential Regulation of NRAMP and IRT Metal transporter genes in wild type and iron uptake mutants of tomato. J Biol Chem. 2003; 278: 24697-24704. DOI: https://doi.org/10.1074/jbc.M301365200
- Sharma SS, Dietz KJ, Mimura T. Vacuolar compartmentalization as indispensable component of heavy metal detoxification in plants. Plant Cell Environ. 2016; 39: 1112-1126. DOI: https://doi.org/10.1111/pce.12706
- Ali H, Khan E, Sajad MA. Phytoremediation of heavy metals - concepts and applications. Chemosphere. 2013; 91: 869-881. DOI: https://doi.org/10.1016/j.chemosphere.2013.01.075
- De Oliveira L, Ma L, Santos J, Guilherme L, Lessl J. Effects of arsenate, chromate, and sulfate on arsenic and chromium uptake and translocation by arsenic hyperaccumulator Pteris vittata L. Environ Pollut. 2014; 184: 187-192. DOI: https://doi.org/10.1016/j.envpol.2013.08.025.
- Koller CE, Patrick WJ, Rose RJ, Offler CE, MacFarlane GR. Arsenic and heavy metal accumulation by Pteris vittata L. and P. umbrosa R. Br. Bull. Environ Contam Toxicol. 2008; 80: 128-133. DOI: https://doi.org/10.1007/s00128-007-9330-4.
- Xun Y, Feng L, Li Y, Dong H. Mercury accumulation plant Cyrtomium macrophyllum and its potential for phytoremediation of mercury polluted sites. Chemosphere. 2017; 189: 161-170. DOI: https://doi.org/10.1016/j.chemosphere.2017.09.055
- Chamba I, Rosado D, Kalinhoff C, Thangaswamy S, Sánchez-Rodríguez A, Gazquez M. Erato polymnioides – A novel Hg hyperaccumulator plant in ecuadorian rainforest acid soils with potential of microbe-associated phytoremediation. Chemosphere. 2017; 188: 633-641. DOI: https://doi.org/10.1016/j.chemosphere.2017.08.160
- Millán R, Gamarra R, Schmid T, Sierra M, Quejido AJ, Sánchez DM, et al. Mercury content in vegetation and soils of the Almadén mining area (Spain). Sci Total Environ. 2006; 368: 79-87. DOI: https://doi.org/10.1016/j.scitotenv.2005.09.096
- Hernández LE, Sobrino-Plata J, Montero-Palmero M, Carrasco-Gil S, Flores-Cáceres M, Ortega-Villasante C, et al. Contribution of glutathione to the control of cellular redox homeostasis under toxic metal and metalloid stress. J Exp Bot. 2015; 66: 2901-2911. DOI: https://doi.org/10.1093/jxb/erv063
- Mendez MO, Maier RM. Phytostabilization of mine tailings in arid and semiarid environments - An emerging remediation technology. Environ Health Perspect. 2008; 116: 278-283. DOI: https://doi.org/10.1289/ehp.10608
- Burkart A. A monograph of the genus Prosopis (Leguminosae subfam. Mimosoideae). Catalogue of the recognized species of Prosopis. J Arnold Arbor. 1976; 57: 450-525.
- Senthilkumar P, Prince WSPM, Sivakumar S, Subbhuraam CV. Prosopis juliflora - A green solution to decontaminate heavy metal (Cu and Cd) contaminated soils, Chemosphere. 2005; 60: 1493-1496. DOI: https://doi.org/10.1016/j.chemosphere.2005.02.022
- Zappala MN, Ellzey JT, Bader J, Peralta-Videa JR, Gardea-Torresdey J. Prosopis pubescens (screw bean mesquite) seedlings are hyperaccumulators of copper. Arch Environ Contam Toxicol. 2013; 65: 212-223. DOI: https://doi.org/10.1007/s00244-013-9904-6
- Mahar A, Wang P, Ali A, Awasthi M, Lahori A, Wang Q, et al. Challenges and opportunities in the phytoremediation of heavy metals contaminated soils: A review, Ecotoxicol. Environ Saf. 2016; 126: 111-121. DOI: https://doi.org/10.1016/j.ecoenv.2015.12.023
- Padmavathiamma P, Li L. Phytoremediation technology: Hyper-accumulation metals in plants. Water Air Soil Pollut. 2007; 184: 105-126. DOI: https://doi.org/10.1007/s11270-007-9401-5
- Thakur S, Singh L, Wahid Z, Siddiqui M, Atnaw S, Din M. Plant-driven removal of heavy metals from soil: Uptake, translocation, tolerance mechanism, challenges, and future perspectives. Environ Monit Assess. 2016; 188: 206. DOI: https://doi.org/10.1007/s10661-016-5211-9.