Alejandro Morales-Bayuelo*, Valentina Perez-Quinones, Zvikomborero Zinhumwe and Praveen Mallri
Volume6-Issue5
Dates: Received: 2025-04-01 | Accepted: 2025-05-07 | Published: 2025-05-10
Pages: 417-432
Abstract
Introduction: The COVID-19 pandemic, an urgent global public health emergency, has resulted in millions of confirmed cases and deaths. Global healthcare systems face significant strain as facilities struggle to manage hospital capacities amid supply shortages. RdRp functions as the essential enzyme for viral replication through respiratory particles. The Food and Drug Administration recognizes Remdesivir as the only approved drug for COVID-19, yet scientists continue to explore additional treatment options.
Methods: The research implemented Glide docking software to perform Standard Precision (SP) and Extra Precision (XP) simulations. The docking grid maintained default parameters for the IFD method, which focused on accommodating different receptor conformations targeting RNA-dependent RNA polymerase's binding pocket. Molecular Quantum Similarity Measure (MQSM) performed Quantum similarity analyses through ZAB quantitative measures that evaluated molecules based on their Density Function (DFs). The molecular quantum similarity (MQS) calculation required two operators: the Dirac delta function and the Coulomb operator. The chemical reactivity analysis involved determining four primary indices, namely chemical potential (µ), hardness (η), softness (S) and electrophilicity (ɷ), to assess ligand stability and electrophilic characteristics alongside reactivity. Fukui functions revealed local chemical reactivity sites of the ligands to provide complete information about their reaction patterns.
Results: The molecular docking analysis showed that ligands formed important bonds with particular residues inside the binding pocket through hydrogen bonds to GLU166 and CYS145. The protein residues develop essential binding interactions that stabilize ligands. The chemical reactivity assessments revealed global reactivity parameters such as each ligand's chemical potential, hardness and softness, and electrophilicity. The analysis with Fukui functions revealed the electrophilic and nucleophilic areas on ligands to understand their reaction behavior and binding process better.
Conclusion: The multi-method research approach integrates molecular docking quantum similarity analysis and chemical reactivity studies and delivers essential details about ligands targeting the SARS-CoV-2 RNA-dependent RNA polymerase. The research findings support crucial advancements in designing drugs for COVID-19 treatment through rational methods.
FullText HTML
FullText PDF
DOI: 10.37871/jbres2099
Certificate of Publication
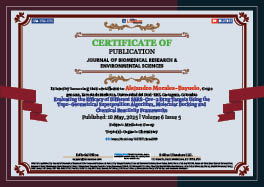
Copyright
© 2025 Morales-Bayuelo A, et al. Distributed under Creative Commons CC-BY 4.0
How to cite this article
Morales-Bayuelo A, Pérez-Quiñones V, Zinhumwe Z, Mallri P. Evaluating the Effi cacy of Different SARSCov- 2 Drug Targets Using the Topo-Geometrical Superposition Algorithm, Molecular Docking and Chemical Reactivity Frameworks. J Biomed Res Environ Sci. 2025 May 10; 6(5): 417-432. doi: 10.37871/jbres2099, Article ID: JBRES2099, Available at: https://www. jelsciences.com/articles/jbres2099.pdf
Subject area(s)
References
1. COVID-19 consulted. 2023.
2. Banerjee A, Kulcsar K, Misra V, Frieman M, Mossman K. Bats and Coronaviruses. Viruses. 2019 Jan 9;11(1):41. doi: 10.3390/v11010041. PMID: 30634396; PMCID: PMC6356540.
3. Yang D, Leibowitz JL. The structure and functions of coronavirus genomic 3' and 5' ends. Virus Res. 2015 Aug 3;206:120-33. doi: 10.1016/j.virusres.2015.02.025. Epub 2015 Feb 28. PMID: 25736566; PMCID: PMC4476908.
4. Song Z, Xu Y, Bao L, Zhang L, Yu P, Qu Y, Zhu H, Zhao W, Han Y, Qin C. From SARS to MERS, Thrusting Coronaviruses into the Spotlight. Viruses. 2019 Jan 14;11(1):59. doi: 10.3390/v11010059. PMID: 30646565; PMCID: PMC6357155.
5. a) Graham RL, Donaldson EF, Baric RS. A decade after SARS: strategies for controlling emerging coronaviruses. Nat Rev Microbiol. 2013 Dec;11(12):836-48. doi: 10.1038/nrmicro3143. Epub 2013 Nov 11. PMID: 24217413; PMCID: PMC5147543. b) Morales-Bayuelo A, Sánchez-Márquez J. Receptor-Based Pharmacophore Modelling of a series of ligands used as inhibitors of the SARS-CoV-2 virus by complementary theoretical approaches, molecular docking, and reactivity descriptors. F1000Res. 2023 Jun 26;12:749. doi: 10.12688/f1000research.133426.1. PMID: 39291142; PMCID: PMC11406136.
6. Burley SK, Berman HM, Bhikadiya C, Bi C, Chen L, Di Costanzo L, Christie C, Dalenberg K, Duarte JM, Dutta S, Feng Z, Ghosh S, Goodsell DS, Green RK, Guranovic V, Guzenko D, Hudson BP, Kalro T, Liang Y, Lowe R, Namkoong H, Peisach E, Periskova I, Prlic A, Randle C, Rose A, Rose P, Sala R, Sekharan M, Shao C, Tan L, Tao YP, Valasatava Y, Voigt M, Westbrook J, Woo J, Yang H, Young J, Zhuravleva M, Zardecki C. RCSB Protein Data Bank: biological macromolecular structures enabling research and education in fundamental biology, biomedicine, biotechnology and energy. Nucleic Acids Res. 2019 Jan 8;47(D1):D464-D474. doi: 10.1093/nar/gky1004. PMID: 30357411; PMCID: PMC6324064.
7. Halgren JJ. Klicic DT. Mainz MP. Repasky EH, Knoll M, Shelley JK, Perry DE, Shaw P, Francis PS, Shenkin. Glide: A new approach for rapid, accurate docking and scor- ing. 1. Method and assessment of docking accuracy. J Med Chem. 2025;47(7):1739-49. doi: 10.1021/jm0306430.
8. Friesner RA, Banks JL, Murphy RB, Halgren TA, Klicic JJ, Mainz DT, Repasky MP, Knoll EH, Shelley M, Perry JK, Shaw DE, Francis P, Shenkin PS. Glide: a new approach for rapid, accurate docking and scoring. 1. Method and assessment of docking accuracy. J Med Chem. 2004 Mar 25;47(7):1739-49. doi: 10.1021/jm0306430. PMID: 15027865.
9. Sastry GM, Adzhigirey M, Day T, Annabhimoju R, Sherman W. Protein and ligand preparation: parameters, protocols, and influence on virtual screening enrichments. J Comput Aided Mol Des. 2013 Mar;27(3):221-34. doi: 10.1007/s10822-013-9644-8. Epub 2013 Apr 12. PMID: 23579614.
10. Jorgensen WL, Maxwell DS, Tirado-Rives J. Development and testing of the OPLS all-atom force field on conformational energetics and properties of organic liquids. J Am Chem Soc. 1996;118:11225-11236. doi: 10.1021/ ja9621760.
11. (a) Carbó-Dorca R, Amat L, Besalú E, Lobato M. Quantum similarity. Adv Molec Simil. 1998.
(b) Carbó-Dorca R, Besalú E. A general survey of molecular quantum similarity huzinaga symposium, fukuoka. J Molec Struct Theochem. 1998.
(c) Solá M, Mestres J, Carbó R, Duran M. A Comparative analysis by means of quantum molecular similarity measures of density distributions derived from conventional ab initio and density functional methods. J Chem Phys. 1996. doi: 10.1063/1.470859.
(d) Carbó R, Besalú E. Theoretical foundation of quantum similarity in "molecular similarity and reactivity: From quantum chemical to phenomenological approaches. In: Carbó R Editor. Understanding chemical reactivity. Kluwer Academic Publishers (Amsterdam). 1995.
12. (a) Morales-Bayuelo A, Matute RA, Caballero J. J Mol Mod. 2015.
(b) Morales-Bayuelo A, Vivas-Reyes R. J Quant Chem. 2014.
(c) Morales-Bayuelo A, Vivas-Reyes R. J Math Chem. 2013.
(d) Morales-Bayuelo A, Valdiris V, Vivas-Reyes R. J Theor. Chem. 2014.
(e) Morales-Bayuelo A, Torres J, Baldiris R, Vivas-Reyes R. Int J Quant Chem. 2012. doi: 10.1002/qua.23288.
(f) Morales-Bayuelo A, Torres J, Vivas-Reyes R. Int J Quant Chem. 2012. doi: 10.1002/qua.23284.
(g) Morales-Bayuelo A, Baldiris R, Vivas-Reyes R. J Theor Chem. 2013. doi: 10.1155/2014/239845.
13. Te Velde G, Velde G te, Bickelhaupt FM, Baerends EJ, Fonseca Guerra C, a van Gisbergen SJ, Snijders JG, Ziegler T, J Comput Chem. 2001.
14. Van lenthe E. J Chem. 1994.
15. Perdew JP, Wang Y. Accurate and simple analytic representation of the electron-gas correlation energy. Phys Rev B Condens Matter. 1992 Jun 15;45(23):13244-13249. doi: 10.1103/physrevb.45.13244. PMID: 10001404.
16. Pye CC, Ziegler T, van Lenthe E, Louwen JN. An implementation of the conductor-like screening model of solvation within the Amsterdam density functional package — Part II. COSMO for real solvents. Can. J Chem. doi: 10.1139/V09-008. 2009.
17. Schipper PRT, Gritsenko OV, van Gisbergen SJA, Baerends EJ. Molecular calculations of excitation energies and (hyper)polarizabilities with a statistical average of orbital model exchange-correlation potentials. J Chem Phys. doi: 10.1063/1.480688. 2000.
18. Carbó-Dorca R, Leyda L, Arnau M. How similar is a molecule to another? An electron density measure of similarity between two molecular structures. Int J Quantum Chem. doi: 10.1002/qua.560170612. 1980.
19. Carbó-Dorca R, Gironés X. Int J Quant Chem. 2005.
20. Bultinck P, Gironés X, Carbó-Dorca R. Reviews in computational chemistry, volume 21. Rev Comput Chem. 2005.
21. Constans P, Amat L, Carbó-Dorca R. J Comput Chem. 1997.
22. Carbó-Dorca R, Mercado LD. Quantum similarity and discrete representation of molecular sets. J Com Chem. 2010.
23. Gironés X, Carbó-Dorca R, QSAR Combinator. Sci. 2006.
24. Carbó-Dorca R, Besalú E, Mercado LD, J Com Chem. 2011.
25. Carbó-Dorca R, Gironés X, Int J Quantum Chem. 2005.
26. Carbó-Dorca R, Besalú E, J Comp Chem. 2010.
27. Morales-Bayuelo A, Ayazo H, Vivas-Reyes R. Europ J Med Chem. 2010.
28. Morales-Bayuelo A, Torres J, Vivas-Reyes R. Quantum molecular similarity analysis and quantitative definition of catecholamines with respect to biogenic monoamines associated: Scale alpha and beta of quantitative convergence. Int J Quant Chem. doi: 10.1002/qua.23284. 2012.
29. Morales-Bayuelo A, Torres J, R. Baldiris R, Vivas-Reyes R. Quantum molecular similarity analysis and quantitative definition of catecholamines with respect to biogenic monoamines associated: Scale alpha and beta of quantitative convergence. Int J Quant Chem. 2012. doi: 10.1002/qua.23284.
30. Morales-Bayuelo A, Torres J, Vivas-Reyes R. Hückel treatment of pyrrole and pentalene as a function of cyclopentadienyl using Local Quantum Similarity Index (LQSI) and the Topo-Geometrical Superposition Approach (TGSA). doi: 10.1142/S0219633612500150. J Theo Comp Chem. 2012.
31. Morales-Bayuelo A, Vivas-Reyes R. Topological model to quantify the global reactivity indexes as local in Diels–Alder reactions, using Density Function Theory (DFT) and Local Quantum Similarity (LQS). J Math Chem. doi: 10.1007/s10910-012-0069-8. 2013.
32. Morales-Bayuelo A, R Baldiris, Vivas-Reyes R. J Theor Chem. 2013.
33. Morales-Bayuelo A, Vivas-Reyes R. J Quant Chem. 2014.
34. Morales-Bayuelo A, V Valdiris, Vivas-Reyes R. J Theor Chem. 2014
35. Morales-Bayuelo A, Vivas-Reyes R. Theoretical calculations and modeling for the molecular polarization of furan and thiophene under the action of an electric field using quantum similarity. J Quant Chem. 2014. doi : 10.1155/2014/585394.
36. Parr RG, Pearson RG. Absolute hardness: Companion parameter to absolute electronegativity. J Am Chem Soc. 1983;105(26). doi: 10.1021/ja00364a005.
37. Geerlings P, De Proft F, Langenaeker W. Conceptual density functional theory. Chem Rev. 2003 May;103(5):1793-873. doi: 10.1021/cr990029p. PMID: 12744694.
38. Chattaraj PK, Sarkar U, Roy DR. Electrophilicity index. Chem Rev. 2006 Jun;106(6):2065-91. doi: 10.1021/cr040109f. PMID: 16771443.
39. Parr RG, Szentpaly Lv, Liu S. Electrophilicity Index. J Am Chem Soc. 1999;121(9). doi: 10.1021/ja983494x.
40. Galván M, Pérez P, Contreras R, Fuentealba P. A direct evaluation of regional Fukui functions in molecules. Chem Phys Lett. 1999;304(5-6):405-413. doi: 10.1016/S0009-2614(99)00325-5.
41. Yang W, Mortier WJ. The use of global and local molecular parameters for the analysis of the gas-phase basicity of amines. J Am Chem Soc. 1986 Sep 1;108(19):5708-11. doi: 10.1021/ja00279a008. PMID: 22175316.
42. Fuentealba P, Pérez P, Contreras R. Higher order derivatives for nuclear indexes in the framework of density functional theory. J Chem Phys. 2000;115:6822-6826. doi: 10.1063/1.1394755.
43. Y 45. Y Zhao, DG Truhlar, The M06 suite of density functionals for main group thermochemistry, thermochemical kinetics, non-covalent interactions, excited states, and transition elements: two new functionals and systematic testing of four M06-class functionals and 12 other functionals, Theor. Chem. Acc. 2008;120:215. doi: 10.1007/s00214-007-0310-x.
44. MJ Frisch, GW Trucks, HB Schlegel, GE Scuseria, MA Robb, JR Cheeseman, G Scalmani, V Barone, B Mennucci, GA Petersson, H Nakatsuji, M Caricato, X Li, HP Hratchian, AF Izmaylov, J Bloino, G Zheng, JL Sonnenberg, M Hada, M Ehara, K Toyota, R Fukuda, J Hasegawa, M Ishida, T Nakajima, Y Honda, O Kitao, H Nakai, T Vreven, JA Montgomery, JE Peralta, F Ogliaro, M Bearpark, JJ Heyd, E Brothers, KN Kudin, VN Staroverov, R Kobayashi, J Normand, K Raghavachari, A Rendell, JC Burant, SS Iyengar, J Tomasi, M Cossi, N Rega, JM Millam, M Klene, JE Knox, JB Cross, V Bakken, C Adamo, J Jaramillo, R Gomperts, RE Stratmann, O Yazyev, AJ Austin, R Cammi, C Pomelli, JW Ochterski, RL Martin, K Morokuma, VG Zakrzewski, GA Voth, P Salvador, JJ Dannenberg, S Dapprich, AD Daniels, O Farkas, JB Foresman, JV Ortiz, J Cioslowski, DJ Fox. Spectroscopic Evaluation of the Molecular Structures of di-μ-Chlorobis(1,5-Cyclooctadiene) Iridium (I) and Rhodium (I) Complexes. Gaussian Inc. Wallingford CT; 2009.