Serhiy P Havryliuk, Ievhenia M Krasnobryzha, Olena S Havryliuk and Heorgii L Volkov*
Volume3-Issue1
Dates: Received: 2022-01-07 | Accepted: 2022-01-13 | Published: 2022-01-17
Pages: 044-059
Abstract
According to "The Proteome Code" concept introduced by J. Biro and our early development of affinity peptide calculation method it was studied the possibility of high affinity peptide chromatographic gels development for IgG1-4 separation from the donor plasma. Given the next step of virus inactivation of IgG directly in the chromatographic column, the affinity gel had bind IgG at several spatially spaced points in order to limit the degree of freedom of the protein for retention IgG at high buffer flow rate or elevated buffer temperatures without denaturation. In addition, the possibility of creating highly specific affinity sense-antisense peptides against Rubella virus in order to increase the titer of aRIgG in plasma or even its isolation in highly purified form was studied. Based on previous experiments, an affinity multi-peptide chromatographic gel with the following properties was developed: the DBC with enough residence time 10 min was around 50-54 mg × mL-1 of total 98.0% purity of IgG with natural proportion of the 1-4 subclasses, any other immunoglobulins were not found. The virus inactivation/elimination on this gel directly in chromatographic column shown a highly effective virus elimination (log10>9) for both nonenveloped and lipid enveloped viruses.
Using RV sequence from UniProt_KB and dates from more than 20 literature sources on the virus proteins interaction, affinity peptides were calculated against virus proteins C and E1,2. Then these peptides were modified to reach more affinity enhancement and affinity-peptide chromatographic gel was synthetized. By this gel from total mass IgG1-4 contained 6644 IU anti-Rubella IgG with specificity 6.64 IU × mg-1 were isolated 5382 IU aRIgG (> 80%) with a specificity of 791 IU × mg-1.
FullText HTML
FullText PDF
DOI: 10.37871/jbres1399
Certificate of Publication
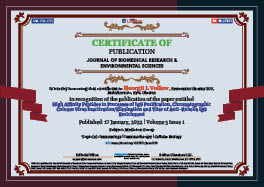
Copyright
© 2022 Havryliuk SP, et al. Distributed under Creative Commons CC-BY 4.0
How to cite this article
Havryliuk SP, Krasnobryzha IM, Havryliuk OS, Volkov HL. High Affinity Peptides in Processes of IgG Purification, Chromatographic Column Virus Inactivation/Elimination and Titer of Anti-Rubella IgG Enrichment. J Biomed Res Environ Sci. 2022 Jan 17; 3(1): 044-059. doi: 10.37871/jbres1399, Article ID: JBRES1399, Available at: https://www.jelsciences.com/articles/jbres1399.pdf
Subject area(s)
References
- Volkov GL, Havryliuk SP, Krasnobryzha IM, Havryliuk OS. The protein/peptide direct virus inactivation during chromatographic process: developing approaches. Appl Biochem Biotechnol. 2017;181(1):233-249. doi: 10.1007/s12010-016-2209-2.
- Havryliuk, SP, Krasnobryzha IM, Havryliuk OS, Volkov GL. The simultaneous human FVIII/vWF purification and virus inactivation combined in chromatographic column. J Biomol Res Ther. 2017;6(2):1000157. doi: 10.4172/2167-7956.1000157.
- Yoshidome T, Kinoshita M. Physical origin of hydrophobicity studied in terms of cold denaturation of proteins: comparison between water and simple fluids. Phys Chem Chem Phys. 2012;14(42):14554-14566. doi: 10.1039/C2CP41738C.
- Risso PH, Borraccetti, DM, Araujo C, Hidalgo ME, Gatti CA. Effect of temperature and pH on the aggregation and the surface hydrophobicity of bovine κ-casein. Colloid Polym Sci. 2008;286(12):1369-1378. doi: 10.1007/s00396-008-1906-y.
- Huang DM, Chandler D. Temperature and length scale dependence of hydrophobic effects and their possible implications for protein folding. PNAS. 2000;97(15):8324-8327. doi: 10.1073/pnas.120176397.
- Van der Vegt, Nayar, D. The hydrophobic effect and the role of cosolvents. J Phys Chem B. 2017;121(43):9986-9998. doi: 10.1021/acs.jpcb.7b06453.
- Mouratou B, Béhar G, Pecorari F. Artificial affinity proteins as ligands of immunoglobulins. Biomolecules. 2015;5:60-75. doi: 10.3390/biom5010060.
- Krepper W, Satzer P, Beyer BM, Jungbauer A. Temperature dependence of antibody adsorption in protein affinity chromatography. J Chromatogr A. 2018;1551:59-68. doi: 10.1016/j.chroma.2018.03.059.
- Alexander P, Fahnestock S, Lee T, Orban J, Bryan P. Thermodynamic analysis of the folding of the streptococcal protein G IgG-binding domains B1 and B2: why small proteins tend to have high denaturation temperatures. Biochemistry. 1992;31(14):3597-3603. doi: 10.1021/bi00129a007.
- Akerström B, Lindahl D, Björck L, Lindqvist A. Protein Arp and protein H from group A streptococci. Ig binding and dimerization are regulated by temperature. J Immunol. 1992;148(10):3238-3243. https://tinyurl.com/hyd2kf8m.
- Franklin JN, Victa C, Donald P, Fahrner R. Fragments of protein an eluted during protein an affinity chromatography. J Chromatogr A. 2007;1163(1-2):105-111. doi: 10.1016/j.chroma.2007.06.012.
- Shimoni J, Gunawan F, Thomas A, Vanderlaan M, Stults J. Trace level analysis of leached protein in bioprocess samples without interference from the large excess of rhMAb IgG. J Immunol Methods. 2009;28;341(1-2):59-67. doi: 10.1016/j.jim.2008.10.015.
- Basle YL, Chennell P, Tokhadze N, Astier A, Sautou V. Physicochemical stability of monoclonal antibodies: A review. J Phar Sci. 2020;109(1):169-190. doi: 10.1016/j.xphs.2019.08.009.
- Biro J. System and method to obtain oligo-peptides with specific high affinity to query proteins. US 8145437 B2. 2012. https://tinyurl.com/yc297svx
- Havryliuk SP, Krasnobryzha M, Havryliuk OS, Volkov GL. A simple, cheap and fast method for calculation of peptides to be used as ligands in affinity chromatography or in other applications in biotech and pharma industry. Am J Pharmacol Ther. 2020;4(1):007-018. doi: 10.3787/ajpt.id20.
- Detmer F, Griesbach J, Sleijpen K, Hazenoot J, Hermans P. Rapid implementation of novel affinity purification. Manufacture of commercial-scale next-generation antibody therapies. Bio Process International. Featured report “Speed to market”. 2019;17(10):12-17. https://tinyurl.com/2dsrc7re
- Muguruma K, Fujita K, Fukuda A, Kishimoto S, Sakamoto S, Arima R. Kinetics-based structural requirements of human immunoglobulin G binding peptides. ACS Omega. 2019;4(11);14390-14397. doi: 10.1021/acsomega.9b01104.
- Toughiri R, Wu X, Ruiz D, Huang F, Crissman JW, Dickey M, Froning K, Conner EM, Cujec TP, Demarest SJ. Comparing domain interactions within antibody Fabs with kappa and lambda light chains. MAbs. 2016;8(7):1276-1285. doi: 10.1080/19420862.2016.1214785.
- Biro JC. Genetic code completed the proteomic code and nucleic acid assisted protein folding. Bio International Convention/Boston Convention and Exhibition Center, USA. 2012.
- Chen X, Li R, Pan Z, Qian C, Yang Y. Human monoclonal antibodies block the binding of SARS-CoV-2 spike protein to angiotensin converting enzyme 2 receptor. Cell Mol Immunol. 2020;17(6):647–649. doi: 10.1038/s41423-020-0426-7.
- Huang RY, Lin MS, Kuo TC, Chen CL, Lin CC, Chou YC. Humanized cOVID‐19 decoy antibody effectively blocks viral entry and prevents SARS‐CoV‐2 infection. EMBO Mol Med. 2021;13(1):e12828. doi: 10.15252/emmm.202012828.
- Dimech W, Arachchi N, Cai J, Sahin T, Wilson K. Investigation into low-level anti-rubella virus IgG results reported by commercial immunoassays. Clin Vaccine Immunol. 2013;20(2):255-261. doi: 10.1128/CVI.00603-12.
- Lyashko: The level of immunization against measles by the end of the year will be 78%. 2020. https://tinyurl.com/2p9evnm6
- Cong H, Jiang Y, Tien P. Identification of the myelin oligodendrocyte glycoprotein as a cellular receptor for rubella virus. J Virol. 2011;85(21):11038-11047. doi: 10.1128/JVI.05398-11.
- Trinh QD, Pham NTK, Takada K, Komine S, Hayakawa S. Myelin oligodendrocyte glycoprotein-independent rubella infection of keratinocytes and resistance of first-trimester trophoblast cells to rubella virus in vitro. Viruses. 2018;10(1):23. doi: 10.3390/v10010023.
- McCarthy M, Lovett A, Kerman RH, Overstreet A, Wolinsky JS. Immunodominant T-cell epitopes of rubella virus structural proteins defined by synthetic peptides. J Virol. 1993;67(2):673–681. doi: 10.1128/jvi.67.2.673-681.1993.
- Volkov G, Andrianov, S., Slominskiy A, Havrylyuk O, Goroshnikova T. Advantages of the EBA method for the first stage chromatography plasma proteins re-purification. 2005.
- Volkov GL, Andrianov SI, Slominskiy AY, Havrylyuk OS, Goroshnikova TV. Advantages of the EBA method for the plasma proteins re-purification obtained by alcohol Kohn method. IX Ukrainian Biochem. 2006;2:142.
- Bradford MM. A rapid and sensitive method for the quantitation of microgram quantities of protein utilizing the principle of protein-dye binding. Anal Biochem. 1976;72:248-254. doi: 10.1006/abio.1976.9999.
- Stoscheck CM. Quantitation of protein. Method Enzymol. 1990;182;50-69. doi: 10.1016/0076-6879(90)82008-p.
- SP Bajaj, AE Schmidt, AM Mathur, K Padmanabhan, D Zhong, M Mastri, PJ Fay, Factor IXa: Factor VIIIa interaction. Helix 330-338 of factor IXa interacts with residues 558-565 and spatially adjacent regions of the A2 subunit of Factor VIIIa. JBC. 2001;276(19):16302-16309. doi: 10.1074/jbc.M011680200.
- Bio-Works user instruction for Work Beads 40 ACT, IN40400010. https://tinyurl.com/str7bxne.
- Gavara PR, Bibi NS, Sanchez ML, Grasselli M, Lahore M. Chromatographic characterization and process performance of column-packed anion exchange fibrous adsorbents for high throughput and high capacity bioseparations. Processes. 2015;3:204-221. doi: 10.3390/pr3010204.
- Volkov GL, Havryliuk SP, Krasnobryzha IM, Zhukova AI, Havryliuk OS. Method for isolation complex of Factor VIII and von Willebrand Factor from blood plasma using gel-exclusion column chromatography, UA 110920, 2016.
- Kaerber G. Contribution to the collective treatment of pharmacological series experiments. Naunyn Schmiedberg's Arch. Exp Pathol Pharmakol. 1931;162:480-483. https://tinyurl.com/3dhe4b5p
- Spearman C. The method of “right and wrong cases” (“constant stimuli”) without Gauss’s formulae. Br J Psyhol. 1908;2:277-282. https://tinyurl.com/32p7rk9p
- Zhang J, Storey KB. Bioplot: An easy-to-use R pipeline for automated statistical analysis and data visualization in molecular biology and biochemistry. Peer J. 2016;4:e2436. doi: 10.7717/peerj.2436.
- Tan LK, Shopes RJ, Oi VT, Morrison SL. Influence of the hinge region on complement activation, C1q binding, and segmental flexibility in chimeric human immunoglobulins. PNAS. 1990;87(1):162-166. doi: 10.1073/pnas.87.1.162.
- Canfield SM, Morrison SL. The binding affinity of human IgG for its high affinity Fc receptor is determined by multiple amino acids in the CH2 domain and is modulated by the hinge region. J Exp Med. 1991;173(6):1483-1491. Doi: 10.1084/jem.173.6.1483.
- Williams RC, Malone CC. Rheumatoid-factor-reactive sites on CH2 established by analysis of overlapping peptides of primary sequence. Scand J Immunol. 1994;40(4):443-456. Ddoi: 10.1111/j.1365-3083.1994.tb03487.x.
- Peterson C, Malone CC, Williams RC. Rheumatoid-factor-reactive sites on CH3 established by overlapping 7-mer peptide epitope analysis. Mol Immunol. 1995;32(1):57-75. doi: 10.1016/0161-5890(94)00122-h.
- Shields RL, Namenuk AK, Hong K, Meng YG, Rae J, Briggs J. High resolution mapping of the binding site on human IgG1 for FcγRI, FcγRII, FcγRIII, and FcRn and design of IgG1 variants with improved binding to the FcγR. JBC. 2001;276(9):6591-6604. doi: 10.1074/jbc.M009483200.
- Yuriev E, Ramsland PA, Edmundson AB. Recognition of IgG-derived peptides by a human IgM with an unusual combining site. Scand J Immunol. 2002;55(3):242-255. doi: 10.1046/j.0300-9475.2002.01032.x.
- Dall'Acqua WF, Cook KE, Damschroder MM, Woods RM, Wu H. Modulation of the effector functions of a human IgG1 through engineering of its hinge region. J Immunol. 2006;177(2):1129-1138. doi: 10.4049/jimmunol.177.2.1129.
- Stavenhagen JB, Gorlatov S, Tuaillon N, Rankin CT, Li H. Fc optimization of therapeutic antibodies enhances their ability to kill tumor cells in vitro and controls tumor expansion in vivo via low-affinity activating Fcγ receptors. Cancer Res. 2007;67(18):8882-8890. doi: 10.1158/0008-5472.CAN-07-0696.
- Keeble AH, Zahra K, Forster A, James LC. RIM21 is an IgG receptor that is structurally, thermodynamically, and kinetically conserved. PNAS. 2008;105(16):6045-6050. doi: 10.1073/pnas.0800159105.
- Richards JO, Karki S, Lazar GA, Chen H, Dang W, Desjarlais JR. Optimization of antibody binding to Fc;RIIa enhances macrophage phagocytosis of tumor cells. Mol Cancer Ther. 2008;7(8):2517-2527. doi: 10.1158/1535-7163.MCT-08-0201.
- Jefferis R. The antibody paradigm: Present and future development as a scaffold for biopharmaceutical drugs, Biotechnol. Genet Eng Rev. 2010;26:1-42. doi: 10.5661/bger-26-1.
- Huang B, Liu FF, Dong XY, Sun Y. Molecular mechanism of the affinity interactions between protein A and human immunoglobulin G1 revealed by molecular simulations. J Phys Chem B. 2011;115(14):4168-4176. doi: 10.1021/jp111216g.
- Lee YC, Tsai KC, Leu S, Wang TJ, Liu CY, Yang Y. Isolation, characterization, and molecular modeling of a rheumatoid factor from a Hepatitis C virus infected patient with Sjögren's syndrome. Scientific World J. 2013;516. doi: 10.1155/2013/516516.
- Mimoto F, Igawa T, Kuramochi T, Katada H, Kadono S, Kamikawa T. Novel asymmetrically engineered antibody Fc variant with superior FcγR binding affinity and specificity compared with afucosylated Fc variant. 2013;5(2):229-236. doi: 10.4161/mabs.23452.
- Diebolder CA, Beurskens FJ, Jong RN, Koning RI, Strumane K, Lindorfer MA. Complement is activated by IgG hexamers assembled at the cell surface. Science. 2014;343(6176):1260–1263. doi: 10.1126/science.1248943.
- Kelton W, Mehta N, Charab W, Lee J, Lee CH, Kojima T. IgGA: A “cross-isotype” engineered human Fc antibody domain that displays both IgG-like and IgA-like effector functions. Chem Biol. 2014;21(12):1603-1609. doi: 10.1016/j.chembiol.2014.10.017.
- Jensen PF, Larraillet V, Schlothauer T, Kettenberger H, Hilger M. Investigating the interaction between the neonatal Fc receptor and monoclonal antibody variants by hydrogen/deuterium exchange mass spectrometry. Mol Cell Proteomics. 2015;14(1):148-161. doi: 10.1074/mcp.M114.042044.
- Foss S, Bottermann M, Jonsson A, Sandlie I, James LC, Andersen JT. TRIM21 – from intracellular immunity to therapy. Front Immunol. 2019;10:2049. doi: 10.3389/fimmu.2019.02049.
- Foss S, Watkinson R, Sandlie I, James LC, Andersen JT. TRIM21: A cytosolic Fc receptor with broad antibody isotype specificity. Immunol Rev. 2015;268(1):328-339. doi: 10.1111/imr.12363.
- Kiyoshi M, Caaveiro JMM, Kawai T, Tashiro S, Ide, T, Asaoka Y. Structural basis for binding of human IgG1 to its high-affinity human receptor FcγRI. Nat Commun. 2015;6:6866. doi: 10.1038/ncomms7866.
- Oganesyan V, Mazor Y, Yang C, Cook KE, Woods RM, Ferguson A. Structural insights into the interaction of human IgG1 with FcγRI: No direct role of glycans in binding. Acta Cryst. 2015;71(Pt 11):2354-2361. doi: 10.1107/S1399004715018015.
- Monnet C, Jorieux S, Urbain R, Fournier N, Bouayadi K, De Romeuf. Selection of IgG variants with increased FcRn binding using random and directed mutagenesis: impact on effector functions. Front Immunol. 2015;6:39. doi: 10.3389/fimmu.2015.00039.
- Irani V, Guy AJ, Andrewa D, Beeson JG, Ramsland PA, Richards JS. Molecular properties of human IgG subclasses and their implications for designing therapeutic monoclonal antibodies against infectious diseases. Mol Immunol. 2015;67(2 Pt A):171-182. doi: 10.1016/j.molimm.2015.03.255.
- Walters, Jensen PF, Larailet V, Lin K, Patapoff T, Schlothauer T. Conformational destabilization of immunoglobulin G increases the low pH binding affinity with the neonatal Fc receptor. JBC. 2016;291(4):1817-1825. doi: 10.1074/jbc.M115.691568.
- Jensen PF, Schoch A, Larraillet V, Hilger M, Schlothauer T, Emrich T, Rand KD. A two-pronged binding mechanism of IgG to the neonatal Fc receptor controls complex stability and IgG serum half-life. Molecular & Cellular Proteomics. 2016;16(3):451-456. doi: 10.1074/mcp.M116.064675.
- Ashoor DN, Ben Khalaf, Hachemi S, Marzouq MH, Fathallah MD. Engineering of the upper hinge region of human IgG1 Fc enhances the binding affinity to FcγIIIa (CD16a) receptor isoform. Protein Engineering Design & Selection. 2018;31(6):205-212. doi: 10.1093/protein/gzy019.
- Shiroishi M, Ito Y, Shimokawa K, Lee JM, Kusakabe T, Ueda T. Structure–function analyses of a stereotypic rheumatoid factor unravel the structural basis for germline-encoded antibody autoreactivity. JBC. 2018;293(18):7008-7016. doi: 10.1074/jbc.M117.814475.
- Wang Х, Mathieu M, Brezski RJ. IgG Fc engineering to modulate antibody effector functions. Protein Cell 2018;9(1):63–73. Doi: 10.1007/s13238-017-0473-8.
- Saunders KO. Conceptual approaches to modulating antibody effector functions and circulation half-life. Front Immunol. 2019;10:1296. doi: 10.3389/fimmu.2019.01296.
- Yogo R, Yamaguchi Y, Watanabe H, Yagi H, Satoh T, Nakanishi M. The Fab portion of immunoglobulin G contributes to its binding to Fcγ receptor III. Sci Rep. 2019;9(1):11957. doi: 10.1038/s41598-019-48323-w.
- Vidarte L, Pastor C, Mas S, Blázquez AB, Rios V, Guerrero R, Vivanco F. Serine 132 is the C3 covalent attachment point on the CH1 domain of human IgG1. JBC. 2001;276(41):38217-38223. doi: 10.1074/jbc.M104870200.
- Panda S, Zhang J, Yang L, Anand GS, Ding JL. Molecular interaction between natural IgG and ficolin - mechanistic insights on adaptive-innate immune crosstalk. Sci Rep. 2014;4:3675. doi: 10.1038/srep03675.
- Mallery DL, McEwan WA, Bidgood SR, Towers G, Johnson CM, James LC. Antibodies mediate intracellular immunity through tripartite motif containing 21 (TRIM21). PNAS. 2010;107(46):19985-19990. doi: 10.1073/pnas.1014074107.
- Foss S, Watkinson RE, Grevys A, McAdam MB, Bern M, Hoydahl LS. TRIM21 immune signaling is more sensitive to antibody affinity than its neutralization activity. J Immunol. 2016;196(8):3452-3459. doi: 10.4049/jimmunol.1502601.
- Bidgood SR, Tam JC, McEwan WA, Mallery DL, James LC. Translocalized IgA mediates neutralization and stimulates innate immunity inside infected cells. PNAS. 2014;111(37):13463-13468. doi: 10.1073/pnas.1410980111.
- Islam N, Shen F, Gurgel PV, Rojas OJ, Carbonell R.G. Dynamic and equilibrium performance of sensors based on short peptide ligands for affinity adsorption of human IgG using surface plasmon resonance. Biosens Bioelectron. 2014;58:380-387. doi: 10.1016/j.bios.2014.02.069.
- Yang H, Gurgel PV, Williams DK, Bobay BG, Cavanagh J, Muddiman DC, Carbonell RG. Binding site on human immunoglobulin G for the affinity ligand HWRGWV. J Mol Recognit. 2010;23(3):271-282. doi: 10.1002/jmr.967.
- Zhao WW, Liu FF, Shi QH, Sun Y. Octapeptide-based affinity chromatography of human immunoglobulin G: Comparisons of three different ligands. J Chromatogr A. 2014;1359:100-111. doi: 10.1016/j.chroma.2014.07.023.
- Kruljec N, Molek P, Hodnik V, Anderluh G, Bratkovič T. Development and characterization of peptide ligands of immunoglobulin G Fc region. Bioconjug Chem. 2018;29(8):2763-2775. doi: 10.1021/acs.bioconjchem.8b00395.
- Williams RC Jr, Malone CC. Rheumatoid-factor-reactive sites on CH2 established by analysis of overlapping peptides of primary sequence. Scand J Immunol. 1994;40(4):443-456. doi: 10.1111/j.1365-3083.1994.tb03487.x.
- Remington KM. Fundamental strategies for viral clearance. Part 2: Technical approaches. BioProcess International. 2015;13(5):10-17. https://tinyurl.com/3hpchs6x
- Alonso WR, Trukawinski S, Savage M, Tenold RA, Hammond DJ. Viral inactivation of intramuscular immune serum globulins. Biologicals. 2000;28(1):5-15. doi: 10.1006/biol.1999.0234.
- Ou D, Chong P, McVeish P, Jefferies WA, Gillam S. Characterization of the specificity and genetic restriction of human CD4+ cytotoxic T cell clones reactive to capsid antigen of rubella virus. Virology. 1992;191(2):680-686. doi: 10.1016/0042-6822(92)90243-i.
- Ou D, Chong P, Tripet B, Gillam S. Analysis of T- and B-cell epitopes of capsid protein of rubella virus by using synthetic peptides. J Virol. 1992;66(3):1674-1681. doi: 10.1128/JVI.66.3.1674-1681.1992.
- Ou D, Chong P, Choi Y, McVeigh, Jefferies WA, Koloitis G, Tingle AJ, Gillam S. Identification of T-cell epitopes on E2 protein of rubella virus, as recognized by human T-cell lines and clones. J Virol. 1992;66(11):6788-6793. doi: 10.1128/JVI.66.11.6788-6793.1992.
- Ou D, Chong P, Tingle AJ, Gillam S. Mapping T‐cell epitopes of rubella virus structural proteins E1, E2, and C recognized by T‐cell lines and clones derived from infected and immunized populations. J Med Virol. 1993;40(3):175-183. doi: 10.1002/JMV.1890400302.
- Wolinsky JS, Sukholutsky E, Moore WT, Lovett A, McCarthy M, Adame B. An antibody- and synthetic peptide-defined rubella virus E1 glycoprotein neutralization domain. J Virol. 1993;67(2):961-968. doi: 10.1128/JVI.67.2.961-968.1993.
- Chaye H, Ou D, Chong P, Gillam S. Human T- and B-cell epitopes of E1 glycoprotein of rubella virus. J Clin Immunol. 1993;13(2):93-100. doi: 10.1007/BF00919265.
- Lovett E, McCarthy M, Wolinsky JS. Mapping cell-mediated immunodominant domains of the rubella virus structural proteins using recombinant proteins and synthetic peptides. J Gen Virol. 1993;74(Pt 3):445-452. doi: 10.1099/0022-1317-74-3-445.
- Marttila J, Ilonen J, Lehtinen M, Parkkonen P, Salmi A. Definition of three minimal T helper cell epitopes of rubella virus E1 glycoprotein. Clin Exp Immunol. 1996;104(3):394-397. doi: 10.1046/j.1365-2249.1996.54762.x.
- Ou D, Mitchell LA, Décarie D, Tingle AJ, Nepom GT. Promiscuous T-cell recognition of a rubella capsid protein epitope restricted by DRB1*0403 and DRB1*0901 molecules sharing an HLA DR supertype. Human Immunol. 1998;59(3):149-157. doi: 10.1016/s0198-8859(98)00006-8.
- Mitchell LA, Tingle AJ, Décarie D, Shukin R. Identification of rubella virus T-cell epitopes recognized in anamnestic response to RA27/3 vaccine: associations with boost in neutralizing antibody titer. Vaccine. 199914;17(19):2356-2365. doi: 10.1016/s0264-410x(99)00040-7.
- Mitchell LA. Sex differences in antibody- and cell-mediated immune response to rubella re-immunisation. J Med Microbiol. 1999;48(12):1075-1080. doi: 10.1099/00222615-48-12-1075.
- Ou D, Mitchell LA, Metzger DL, Gillam S, Tingle AJ. Cross-reactive rubella virus and glutamic acid decarboxylase (65 and 67) protein determinants recognised by T cells of patients with type I diabetes mellitus. Diabetologia. 2000;43(6):750-762. doi: 10.1007/s001250051373.
- Robinson K, Mostratos A, Grencis RK. Generation of rubella virus-neutralizing antibodies by vaccination with synthetic peptides. FEMS Immunol Med Microbiol. 1995;10(3-4):191-198. doi: 10.1111/j.1574-695X.1995.tb00033.x.
- Beatch MD, Hobman TC. Rubella virus capsid associates with host cell protein p32 and localizes to mitochondria. J Virol. 2000;74(12):5569-5576. doi: 10.1128/JVI.74.12.5569-5576.2000.
- Ghebrehiwet B, Atreya, CD. The N-terminal conserved domain of rubella virus capsid interacts with the C-terminal region of cellular p32 and overexpression of p32 enhances the viral infectivity. Virus Res. 2002;85(2):151-161. doi: 10.1016/s0168-1702(02)00030-8.
- Everitt JC, Law LJ, Hobman TC. Interactions between rubella virus capsid and host protein p32 are important for virus replication. J Virol. 2005;79(16):10807-10820. doi: 10.1128/jvi.79.16.10807-10820.2005.
- Chaye H, Chong P, Tripet B, Brush B, Gillam S. Localization of the virus neutralizing and hemagglutinin epitopes of E1 glycoprotein of rubella virus. Virology. 1992;189(2):483-492. doi: 10.1016/0042-6822(92)90572-7.
- Mitchell LA, Zhang T, Ho M, Décarie D, Tingle AJ, Zrein M, Lacroix M. Characterization of rubella virus-specific antibody responses by using a new synthetic peptide-based enzyme-linked immunosorbent assay. J Clin Microbiol. 1992;30(7):1841-1847. doi: 10.1128/jcm.30.7.1841-1847.1992.
- Cordoba P, Grutadauria SL, Cuffini C, Zapata MT. Presence of a neutralizing domain in isolates of rubella virus in Cordoba, Argentina. Clin Diagn Lab Immunol. 1997;4(4):493-495. doi: 10.1128/cdli.4.4.493-495.1997.
- Cordoba P, Lanoel A, Grutadauria S, Zapata M. Evaluation of antibodies against a rubella virus neutralizing domain for determination of immune status. Clin Diagn Lab Immunol. 2000;7(6):964-966. doi: 10.1128/CDLI.7.6.964-966.2000.
- Elgenaid SN, Hajj EM, Ibrahim AM, Essa ME, Hassan MA. Prediction of multiple peptide based vaccine from e1, e2 and capsid proteins of rubella virus: An in-silico approach. Immunome Res. 2018;14(1):1-13. doi: 10.4172/1745-7580.1000146.